Electrochemomechanical Simulations of 3D-Resolved Solid-State Lithium-Ion Battery Cells
Solid-state batteries have emerged as a viable alternative to traditional liquid-based lithium-ion batteries, offering improved cost efficiency, safety, and environmental impact. Chlorine-rich lithium argyrodite (Li6PS5Cl) has emerged as a promising solid-state electrolyte, presenting high ionic conductivity, excellent compatibility with lithium metal anodes, and electrochemical stability. However, its implementation still poses several challenges, such as complex electrochemical processes, interfacial reactions, and structural integrity. Numerical simulations represent a key tool to optimize battery cells microstructure by providing insights into their performance and durability: in this context, this work presents a simulation protocol for solid-state cell at the microscale level, aiming to assist the cell design process.
The simulation setup was built following the experimental manufacturing parameters and procedures used for laboratory-level argyrodite-based coin cells. Specifically, the anode was modeled as a lithium plate; the cathode was represented by a composition of NMC811, SuperP aggregates, a conductive carbon-based additive, and argyrodite. Finally, the electrodes were separated by a thick layer of argyrodite. A representative cathode geometry was created using a custom open-source code: NMC spheres were packed using a fall-and-roll approach until the particle size distribution provided by the manufacturer was achieved. Instead, the SuperP particles were randomly distributed on the NMC surface, while argyrodite was treated as a homogeneous medium. The generated text files containing the NMC and SuperP coordinates were imported into the COMSOL Multiphysics® software and used within a Method script to automatically build a representative portion of the cell. As the complexity of the cathode structure required a large number of objects (3000+ spheres), the CAD Import Module was used to ease the geometry generation.
The cell performance was simulated considering the coupled interactions of electrochemistry (via the Battery Design Module), lithium transfer within the NMC, heat transfer, and solid mechanics. To achieve this, charge conservation in the NMC, solid electrolyte, and SuperP domains was solved to determine the cell potential. Lithium concentration within the NMC was evaluated through a convection-diffusion equation, coupled with the electrochemical and mechanical model to account for lithium intercalation and stress-induced diffusion, respectively. A 1D model of the coin cell tested was employed to evaluate the cell temperature during operation, which was then used to update the material transport properties. Finally, the displacement field was evaluated using a quasi-static approach and used to investigate the average cell deformation and the potential occurrence of damage.
The 3D-resolved model provided comprehensive insights into the cathode microstructure and cell behavior under various operating conditions. Electrochemical performance was assessed by applying currents ranging from C/20 to 1C; additionally, the electrolyte tortuosity and average cathode electrical conductivity were evaluated. In future work, these results can be used to calibrate 1D and 0D models, facilitating the evaluation of battery performance over extended cycles and incorporating aging effects. In conclusion, the developed numerical framework presents a novel and accurate contribution towards the advancement of affordable and safe lithium-ion batteries, with the goal of supporting the electric transition.
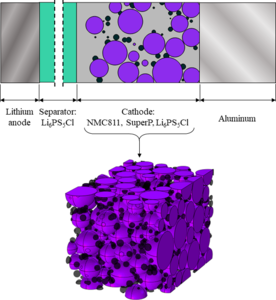