H2SO4 Catalysis: Perspective and Opportunities for Reducing SO2 Emissions
Introduction: Development of next-generation chemical processes that have zero emissions is a key environmental objective for sustainable development. The manufacture of H2SO4 by the air oxidation of SO2 to SO3 is an important technology where an opportunity exists for new catalyst development and process innovation by reducing emissions of unconverted SO2 in process reactor tail gases owing to the sheer number and scale of typical plants [1]. Emissions control technologies using new catalyst technology, improved reactor designs, and process strategies have notable potential. The primary objective of this study is two-fold: (1) to review current particulate H2SO4 catalysts utilized in the oxidation of SO2 to SO3; and (2) to develop a COMSOL Multiphysics® simulation to compare the effect of species flux model on catalyst performance for typical process conditions.
Methods: COMSOL software is used to model the effect of different species flux models on transport-kinetic interactions in various particulate catalyst geometries. This builds upon our previous work on modeling of transport-kinetic interactions in SO2 oxidation catalysts shapes [2]. Various diffusion flux models, namely, the Wilke model, the Wilke-Bosanquet, and the Dusty Gas model are not available in COMSOL so these were coded to compare the particle concentration and temperature profiles as well as the particle effectiveness factor over typical process conditions encountered in multi-pass convertor operation. The reaction kinetic model is based upon the work of Collina et al. [3] since it accounts for the dependence of the SO2 oxidation rate on the partial pressures of SO2, O2, and SO3. The Chemical Reaction Engineering Module is used to evaluate adiabatic reactor performance under ideal reactor conditions.
Results and Discussion: Typical adiabatic 4-pass converter profiles are shown in Figure 1. These are based upon the Chemical Reaction Engineering Module to describe adiabatic reactor performance with ideal plug flow of the gas. These results provide the incentive for developing new catalyst technology because the maximum SO2 conversion possible is 99.7%, which is adequate to meet current EPA regulations for SO2 emissions. However, it does not meet the anticipated future need to design H2SO4 plants with SO2 emissions < 100 ppm, or even < 10 ppm.
Additional results that compare the performance of particulate catalyst shapes using typical process conditions will be presented and discussed, thereby showing the incentive for development of alternate catalyst forms with higher activity and reduced emissions.
Conclusions: COMSOL software provides a convenient platform for quantifying detailed transport-kinetic interactions in commercial catalyst particles having complex shapes. The model-predicted results provide a realistic basis for comparison to experimental data. The results show that the observed catalyst performance is notably affected by the choice of diffusion flux model.
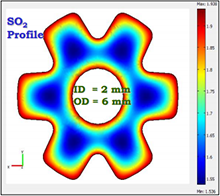
下载
- mills_presentation.pdf- 4.64MB
- mills_abstract.pdf- 0.08MB