Simulation of the Arc Motion in Low-Voltage Surge Protection Devices
Nowadays, surge protection devices are widely used in electrical grids to suppress over voltages during transient conditions caused by voltage surges and to prevent damages of industrial or household devices. The working principle is based on the initiation of an arc with a burning voltage lower than the rated supply voltage. The ignited arc has to be led to its extinction clearing the subsequent over current and avoiding that it is further supplied by the power grid. This can be done by a considerable increment of the electric field of the arc due to arc elongation, wall cooling, arc splitting, magnetic blown-off or a combination of them. The arc development is accompanied by interrelated electromagnetics, fluid flow, and heat transfer and can be characterized as a multiphysics problem. COMSOL Multiphysics® with its capability of implementing complex geometries and using highly efficient solvers and parallel computing is considered for solving this multiphysics problem. The CFD Module, the AC/DC Module and the Heat Transfer Module along with user defined features related to radiative heat transfer, event definition of the arc ignition are used. The arc is ignited in air at atmospheric pressure and the arc plasma is usually considered to be in the state of local thermodynamic equilibrium. A magneto-hydrodynamic model is developed based on the time-dependent partial differential equations for conservation of mass, momentum and energy along with the equations of current continuity, Maxwell equations, Ohm’s law and the equation of state. A very large computation scale remains still a common problem in three-dimensional models. The computational effort can be significantly reduced for simulations, where the arc chamber is slim and does not exhibit substantial irregularities in the third dimension. Models of low-voltage switching devices with parallel or diverging runners can be set up using two-dimensional planar chamber geometries. In this contribution, we show that three-dimensional effects that cause the arc movement can also be considered utilizing a two-dimensional approach. The predicted temporal behaviour of the arc in the chamber is shown in Fig. 1. The distribution of the plasma temperature is shown as obtained from the two-dimensional planar model on the left-hand side and in the plane of symmetry of the 3D model on the right-hand side of the figure. Four instants are chosen to represent the ignition, the distribution at maximum current, and those at 50% and 25% of the maximum during current decay, respectively. The evolution of the arc obtained in both models is very similar. The arc motion occurs in the same way in both models. The computational domain is finer spatially resolved in the two-dimensional model. This allows us to obtain finer details of the arc attachment on the electrodes and towards the gas outflow. The results correlate with the measurements obtained from optical emission spectroscopy. The two-dimensional model is computationally very efficient and is used for preliminary developments, where the influence of changes in the chamber geometry on the arc behaviour can be studied avoiding long times of computation.
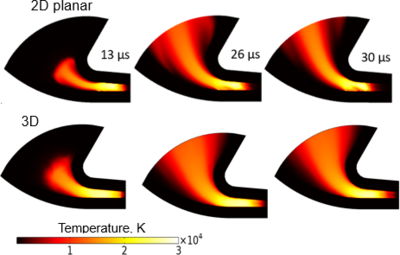
下载
- Baeva_5991_presentation.pdf- 1.14MB
- Baeva_5991_poster.pdf- 0.84MB