Thermo-rheological modelling of the Yellowstone caldera: insights into volcanic processes.
The Yellowstone Volcanic Complex (YVC) in Yellowstone National Park (Wyoming, USA) attracts intense geological interest, as it ranks as one of the largest active continental silicic volcanic fields worldwide. Despite extensive research on the region's high heat flow and abundant geothermal features, a detailed quantitative analysis of the brittle-ductile transition has been lacking. Such analysis is essential for investigating the volcanic plumbing system, advancing geothermal exploration, and improving the assessment of volcanic hazards. This study aims to deepen understanding of the subsurface geological and geophysical properties of the Yellowstone area, with a specific focus on obtaining an optimized lithospheric thermal profile, which is fundamental for reliable rheology and lithospheric strength analysis. Initially, extensive mapping of the Curie isothermal surface depth was performed using high-resolution aeromagnetic data and innovative spectral analysis techniques. This mapping revealed a notably shallow Curie isothermal surface, ranging from 2 km to 4 km deep beneath the YVC. The retrieved depth of the iso-Curie surface was subsequently used as an important constraint to validate the provided 3D stationary Finite Element (FE) thermal model. In particular, we consider this isothermal surface as experimental data for the optimization process of the thermal state of the crust by using the Optimization Module in COMSOL Multiphysics® 6.2. The 3D model of the Yellowstone lithosphere extends over an area of about 40.000 km2, with a lithospheric thickness of about 45 km. The entire domain is subdivided into three litho-thermal units: the upper crust, the lower crust, and the magmatic body. The geometry of the magmatic heat source was derived from tomographic data, specifically from a study on local variations in Vs velocity. This 3D geometry was then incorporated into COMSOL Multiphysics® using the CAD Import Module to generate a consistent subsurface image of the local magmatic heat source. The same functionality was used to introduce the DEM representing the topographic surface of the area. A custom tetrahedral mesh was created, resulting in a volume with 675.906 tetrahedral elements. The thermal state of the crust is simulated using the Heat Transfer in Solids Module in a purely conductive regime. The temperature is fixed at both the top and bottom of the model, with the latter set at 1200°C. The model temperature at the topographic surface varies as altitude function, starting from an average value of 17°C for the whole area. The thermal conductivity of the model materials k(T) was introduced as a temperature-dependent function. No thermal exchange occurs through the lateral boundaries of the 3D domain (Figure 1). A comprehensive rheological model was also conducted to delineate the distribution of the brittle-ductile transition within the analyzed lithospheric volume, by producing specific scripts in the Matlab environment. The results highlight a brittle region that aligns well with the earthquakes distribution and reveal a non-homogeneous structure in ductile zones, supporting the "sandwich hypothesis" for the local lithospheric structure. We conclude that our approach contributes to a better understanding of the YVC subsurface dynamics, providing insights into its complex geodynamic processes and offering methodologies applicable to similar studies in volcanic and geothermally active regions with large calderas.
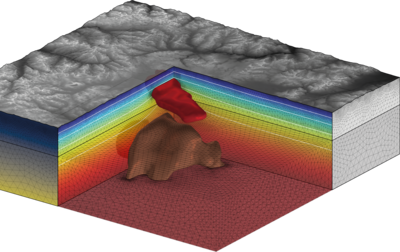
下载
- perrini_9431_poster.pdf - 5.38MB
- 1_maddalena_perrini.pdf - 2.79MB