Topology Optimization of Lithium-Ion Battery Electrode Microstructure Morphology for Reduction of Damage Accumulation and Longevity of Battery Life
The ubiquitous commercial use of Lithium-Ion batteries (LIBs) has increased interest in their implementation into efficient energy storage systems for clean and renewable power sources and the electrical transportation industry. Unfortunately, LIBs are not yet technological mature to meet various commercial demands. Consider the following challenges for example,: (1) lower than desired effective traveling distance due to limited LIB capacity, (2) unsuitable LIB lifespan and (3) relatively high maintenance and battery replacement costs; collectively these issues make electrical vehicles impractical for large-scale consumer production. A major contributor to this bottle-neck in development is battery degradation mechanisms, specifically multiphysics fracture/damage of electrode active materials for intents and purposes. Importance of understanding damage evolution stems from the notion that damage is attributed to causing irreversible capacity loss and limited LIB longevity. Therefore, understanding this phenomenon allows for methods of increasing effective capacity, battery life and reducing damage formation. This presentation will therefore address the development of an advanced multiphysics model, brittle material, continuum damage scheme, and understanding the effects of micro-structure morphology on damage generation in LIB Silicon anodes.
To model the coupled electrochemical and solid mechanical physics of the LIB, the mechano-diffusion governing equations (Figure 1) are implemented in a COMSOL Multiphysics® simulation through equation-based modeling. The General Form PDEs are coupled through the mechanical fields (stress, strain, displacement) and the diffusion field (concentration). To appropriately model the damage accumulation of the system, the evolution law (Figure 1) is implemented through Domain ODEs and DAEs, specifically Distributed ODEs to capture the maximum damage quantity over a desired time interval. Due to the continuity of the finite element implementation within COMSOL, the greater benefits of parallel computing comes from the use of the Cluster Computing study node in convergence studies and the use of the Cluster Sweep study node in parametric and more advanced shape optimizations (in future works).
Thus far, an isotropic continuum damage evolution law derived from Weibull's distribution formulation was coupled with mechano-diffusion physics within COMSOL. Inclusion of anisotropy yields marginal effects on the mechano-diffusion responses (Figure 2) but it is expected that for continuum damage laws there will be significant effect of the behavior of damage evolution dependent of the specific crystal orientation. Future-works will illustrate the effects of shape optimization on stress and damage generation for models of 2D and 3D periodic micro-structure morphologies as seen in Figure 3.
Evidently, study shows that modeling materials such as cubic crystal silicon does not significantly benefit from inclusion of anisotropy in the mechano-diffusion governing equations. This is due to the marginal anisotropic elastic properties and isotropic diffusion of crystal silicon. The effects of anisotropy are however expected to have great influence on the development of multi-phases of silicon, anisotropic morphology changes and their influence on damage evolution which will all be addressed in future works. Implementing topology optimization schemes in future works is expected to aid in new design methods to increase resilience of highly stressed electrode surfaces. This will subsequently increase electrode effective capacity and overall LIB performance for further commercial applications.
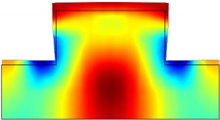
下载
- clarke_presentation.pdf- 2.06MB
- clarke_abstract.pdf- 0.51MB